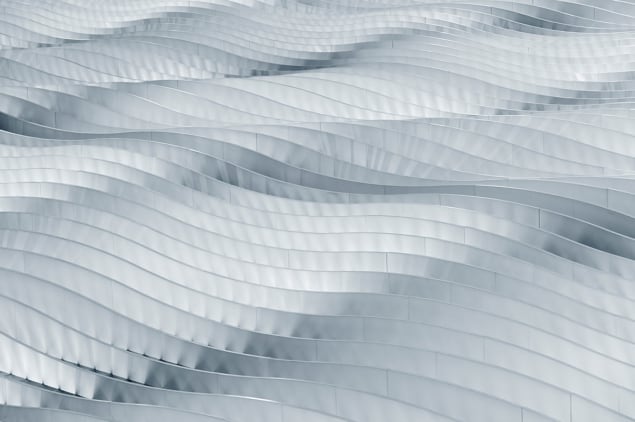
The most important function for an electronic circuit is the off switch. The trillion-dollar global semiconductor industry hinges on the way transistors can switch or amplify signals in integrated circuits – no wonder then that researchers are so interested in finding new ways of creating switches using emerging materials.
Now a collaboration of researchers in the US has demonstrated ultrafast switching in topological Weyl semimetal phases using terahertz light. As well as opening the door to potential applications exploiting these topological phases – such as dissipationless electronics and fault-tolerant quantum computers – the approach could be used to stabilize non-equilibrium topological phases in materials where this kind of behaviour is not otherwise observed.
Topological gets topical
Topological materials can be insulating in the bulk but have conducting surface states due to symmetry-protected topological order. In 1987 physicists in the Soviet Union predicted the existence of time-reversal symmetry-protected edge states, but interest really exploded with the reports of experimentally observed topological insulators in the 2000s. As well as insights into fundamental physics, the topological states are protected from environmental fluctuations and forbid backscatter, the main dissipating process in electronics. These properties could give topological materials significant advantages for next-generation electronic devices, particularly if there is a means of switching them from one state to another.
Aaron Lindenberg, a researcher at SLAC National Accelerator Laboratory and Stanford University in the US and his colleagues focused their attention on a type of “Weyl metal” – exotic materials in their own right that have excitations in the form of massless chiral quasiparticles. Weyl metals are a solution of the Dirac equation where Lorentz symmetry (a central tenet of Einstein’s special theory of relativity) must be maintained, and they play a crucial role in quantum field theory and the Standard Model of particle physics. However, in the context of condensed matter research, Lorentz symmetry is no longer an issue, and generalizing the solution leads to “type II” Weyl semimetals.
Performing under strain
The layered transition-metal dichalcogenide WTe2 that Lindenberg and collaborators studied crystallizes into a distorted lattice with a lack of inversion symmetry that leads to semimetal behaviour with type II Weyl points. As the researchers point out in their report, lattice strain can tune the topological invariants providing a possible route to switching the phase. However, the usual means for applying strain – heteroepitaxial lattice mismatch and dislocations – do not lend themselves to dynamical processes. Instead Lindenberg and team showed that they could apply sufficient lattice strain for ultrafast switching between phases using terahertz light.
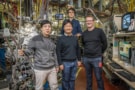
Topological off-on switch could make new type of transistor
Mark Edmonds at Monash University in Australia alongside collaborators in Asia, the US and Europe had previously demonstrated switching between topological phases in ultrathin Na3Bi using an applied electric field to get around the challenges of controlling mechanical strain. In their report Lindenberg and team point out, “Lattice strain provides the most natural means of tuning these topological invariants because it directly modifies the electron–ion interactions and potentially alters the underlying crystalline symmetry on which the topological properties depend.”
The terahertz beam they use couples to the WTe2 exerting a 1% shearwise displacement between adjacent layers. Most materials would fracture under this much strain, but the weak interlayer van der Waals forces in the WTe2 make it less susceptible to damage. As Lindenberg and collaborators explain in their report, the displacement is more than enough to bring Weyl points of opposing chirality together so that they annihilate. However, displacing the layers in the opposite direction could also double the distance between Weyl points so that the topological phase is more robust. As a result, the approach may help diversify the range of available topological materials by stabilizing non-equilibrium topological phases in otherwise trivial materials.
Full details are reported in Nature.